Planétologie de laboratoire et matière dense et tiède
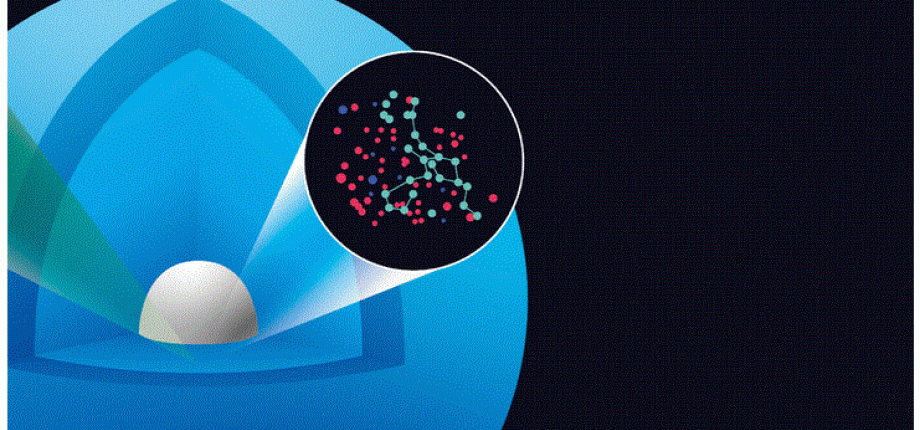
Warm Dense Matter: planetary science and fundamental physics at LULI
The advent of high-power lasers has opened unprecedented opportunities to explore extreme pressures (several Mbar) and temperatures (multi kK), leading to investigations of complex states of matter at the intersection of condensed matter and plasma physics, commonly known as warm dense matter (WDM). Despite its significance in various scientific disciplines, such as Inertial Confinement Fusion (ICF), geophysics, planetology and astrophysics as well as fundamental physics and material science, the theoretical description of WDM remains a formidable challenge, and is still limited.
Planetary science: WDM is of paramount importance in planetary science, as conditions at planetary interiors lie in this regime. Understanding how materials behave under extreme pressure and temperature conditions is essential to refine models of planetary interiors, elucidating their structure, formation, and evolution. This becomes increasingly intriguing today with the continuous discovery of exoplanets. Our research at LULI involves employing laser-based dynamic compression experiments to measure material properties at these conditions. We use optical probes for measuring the equation of state material and electronic conductivity, employing reflectivity, as well as advanced x-ray sources like laser generated x-ray sources, free electron lasers or synchrotrons for microscopic investigations. Among different approaches, our research focuses on “planetary ice” relevant for ice giants planets and iron, iron alloys and silicates for terrestrial planets.
Ice Giant planets: Today, our modelling of Uranus and Neptune is heavily hampered by the poor knowledge of the “planetary ice”, presumably the major component of their mass. This also impacts our ability in charactring the wide set of Neptune-size exoplanets discovered nowadays. In spite of the misleading name, “planetary ices” are complex mixtures of water, ammonia and methane whose properties at high pressure and temperature remain poorly known. Ab initio calculations predict they undergo very peculiar chemical and physical processes at high pressures, including exotic polymerisation, phase separation and the formation of superionic lattices. The existing experimental works are scarce, limited to low pressures and therefore not sufficient to furnish adequate support for theory. At LULI, we have initiated a research activity aiming at characterising the properties Of H2O-NH3-CH4 Mixtures relevant for Planets and (Exo)-planets Interiors. We have successfully designed and fabricated pertinent targets for laser compression techniques, which was one of the main technical challenges that limited the mixture investigation. This allowed us to lead unique experiments. We demonstrated the necessity for planetary models to go beyond the simplistic approach using water as a sole component of the ice giants mantles [M. Guarguaglini et al Sci. Rep. 9, (2019)]. We have also provided the first experimental demonstration of metallic ammonia [A. Ravasio et al. Phys. Rev. Lett. 126, 025003 (2021).] and melting of super-ionic ammonia [J. A. Hernandez et al. Nature Physics, 2023, 19 (9)]. To go beyond optical probes, we have initiated new experiments on XFEL facilities (LCLS experiment #LW03 & EuropeanXFEL experiment #4463) to get direct insights of the microscopic structure and chemical processes occurring in planetary ices. While for water we found lattices in the pressure/temperature regimes typical of super-ionic phases, the first results on CHNO mixtures evidence a richer chemistry.
Terrestrial planets: The study of Earth and earth-like planets deserves a special interest in planetary research because of their close link to the fantastic opportunity to find possible habitable worlds. In the most current description, Earth-like planets are assumed to be fully differentiated and constituted of an iron core surrounded by a silicate mantle. Structural, thermal and transport properties of these compounds at high pressures are paramount to comprehend planetary formation and evolution. For example, the metallisation of liquid silicates under extreme conditions would promote part of the mantle to a potential magnetic field generator. On the other hand, iron melting curve at planets’ core conditions constrains the thermodynamics governing the planet’s heat budget, such as the heat flux from the core to the mantle, the planetary cooling rate and the power available for the geo-dynamo. Our activity at LULI addresses several of the most important questions in modern planetology.
Can SiO2 Magma Oceans Support Magnetic Dynamos? :The interiors of super-Earths are expected to be significantly hotter and experience higher pressures than Earth, potentially leading to the melting of silicate minerals in their mantles and the persistence of magma oceans. To contribute to this understanding, LULI conducted experiments characterising the equation of state and electrical conductivity of SiO2 at Mbar and a few thousand K using the double-shock technique [M. Guarguaglini et al. Nat. Comm. (2021)]. The results, supported by experimental reflectivity data and numerical simulations, revealed high electrical conductivities, suggesting the likelihood of dynamo processes in the mantles of super-Earths. These findings have implications for the habitability of super-Earths and challenge simplistic models of conductivity behaviour under high pressure [M. Guarguaglini et al. Phys. Plasmas (2019)] and highlighted by Ecole Polytechnique: https://www.polytechnique.edu/actualites/comprendre-lespace-en-laboratoire
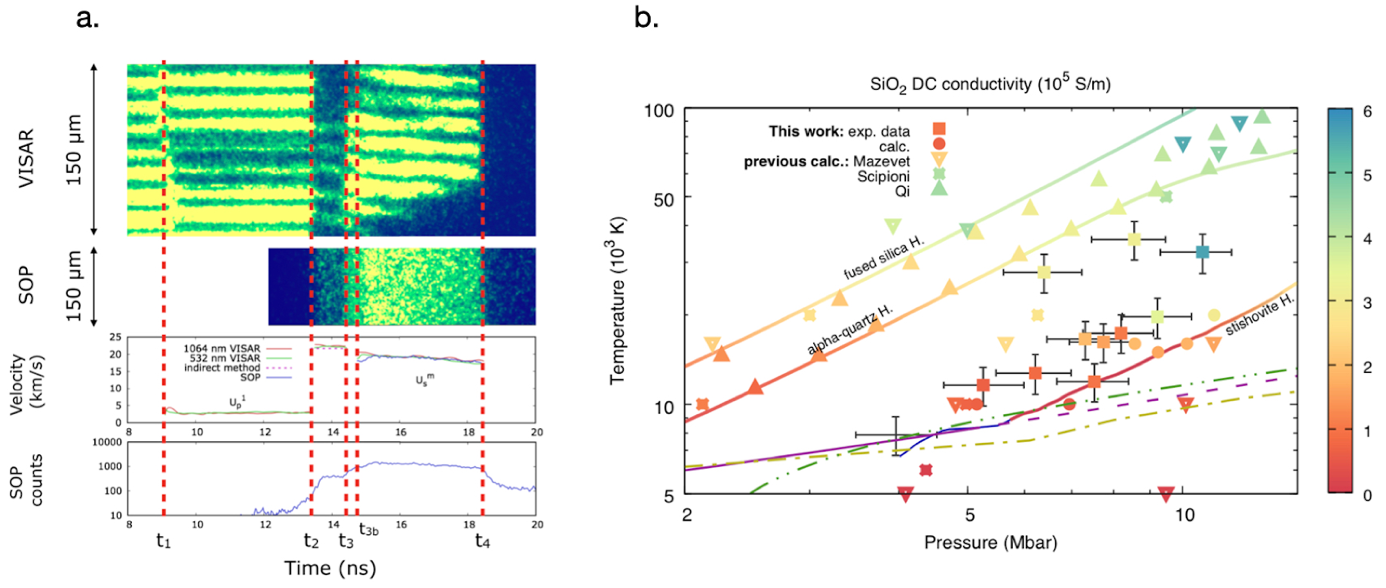
(Mg,Si,O) Systems at Earth’s mantle conditions: MgSiO3 and Mg2SiO4 are crucial components of the mantles of terrestrial planets, and understanding their behaviour under extreme conditions is essential. Laser-driven shock waves were employed at LULI to simulate conditions similar to the deepest part of the early Earth mantle, providing pioneering results on equations of state [Bolis GRL 2015]. Reflectivity measurements at multiple wavelengths (532nm, 1064 nm and 355 nm) have recently been developed. Applied to Fe-free and Iron bearing silicates, it allows to address the impact of Fe on conductivity and gap closure. These data also indicate indirect hints of phase transitions [Bolis GRL 2015]. To get more direct investigations of these transformations, we have performed new experiments using advanced x-ray sources from XFEL. The shock compression of SiO2 quartz, MgSiO3 enstatite crystals and Mg2SiO4 olivine were probed using intense X-ray pulses from the Linac Coherent Light Source XFEL facility. While for SiO2 we found reconstructive phase transformations into crystalline high-pressure polymorphs, for more complex systems MgSiO3 the data revealed unexpected disordered structures instead of expected crystalline phases. This highlights the importance of considering the degree of polymerization of silicates in understanding shock thermodynamics. Similar studies on MgSiO3 glass reinforced the assumption that silicate glasses serve as appropriate structural analogs for understanding the atomic arrangement of silicate liquids at high pressures [J.-A Hernandez et al. Geophys. Res. Lett. (2020)] and [G. Morard et al. PNAS (2020)].
On a similar set-up, we have developed emission spectroscopy (XES) from shocked Mg2SiO4 olivine, for a direct measurement of the Fe spin state at high pressure conditions. These challenging results show dominantly low-spin Fe and supports gravitationally stable melt in the deep mantle and generation of a dynamo from the silicate melt portion of rocky planets [Shim et al. Sci. Adv. (2023)].
On another note, experiments using XANES diagnostics coupled to shock compression on MgO have been carried out at LULI successfully [Bolis2019] putting in evidence changes in electronic structure due to metalization and into the liquid.
Fundamental physics and material science at high pressures: Besides activities with direct implications to planetology, the LULI team is also involved in investigations related to more fundamental physics and materials science. In addition to macroscopic characterization ( e.g. EOS, reflectivity data), an effort has been dedicated to microscopic studies. You can find some examples below.
Phase transitions and atomic structural changes: To complement XFELs, LULI developed in collaboration with CEA an ultrafast X-ray powder diffraction setup (see figure below) for laser-driven dynamic compression at the LULI2000 laser facility. This development facilitates structural measurements, such as the bcc–hcp transition in shock and ramp-compressed polycrystalline iron [A. Denoeud et al. Rev. Sci. Instrum. (2021)]. Recent experiments have shown the recrystallization of GeO2 and metallic glasses under shock. From this research two different experiments have been accepted at LMJ in Bordeaux where a diffraction box similar to the one used at LULI has been developed and will be used to probe materials at extreme pressures.
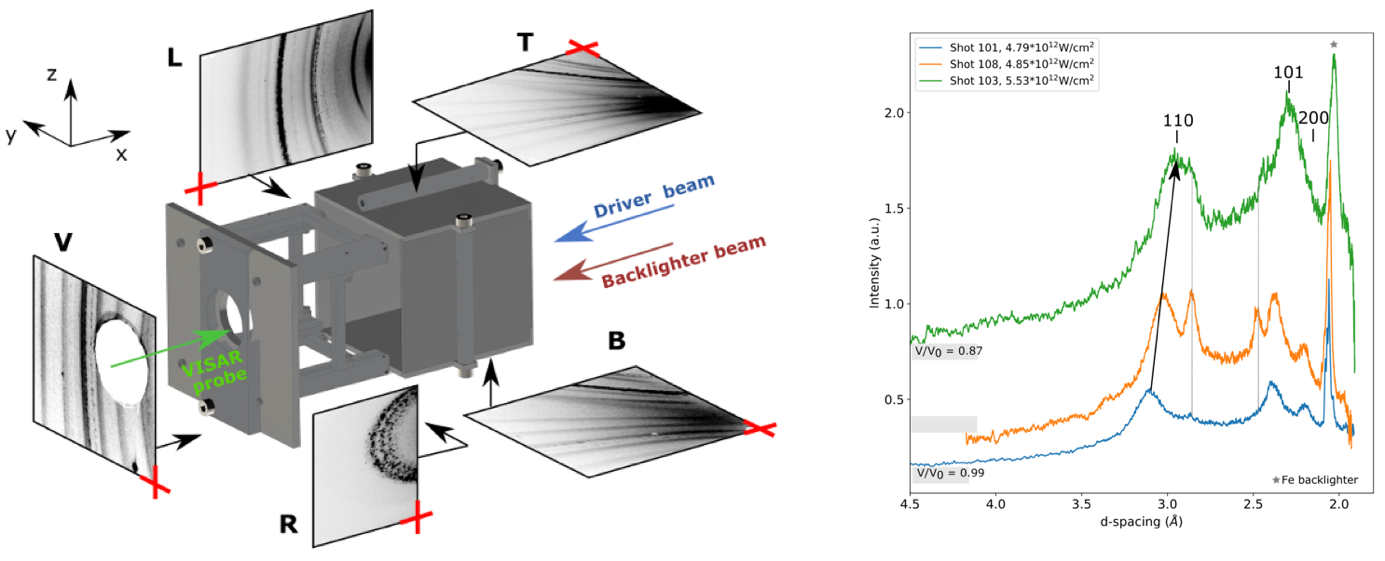
Investigations on electronic structural changes: X-ray Absorption Near Edge Structure (XANES) offer a powerful tool to investigate the electronic structure and chemical composition of materials by monitoring changes in the local environment of atoms in processes such as phase transitions, which occur under dynamic compressions. At LULI, we have explored the liquid structure of MgO [R. Bolis et al. PoP (2019)], but today HPLF at ESRF is the ideal facility to perform high quality studies. The LULI has a strong collaboration with the ESRF team with which he performed interesting studies on copper, iron and on the gap closure mechanism of the GeO2. These results are a strong test bed for approximation used in the ab initio calculations.
Studies of interest for material science: In this context, we've conducted campaigns in strong collaboration with Rennes University on the equation of state of various metallic glasses and their responses to different strain rates using squared and ramped laser pulses available on LULI 2000 [Y. Raffay et al. Int. J. of Impact Engineering (2023)]. Indeed, these materials are considered the materials of the future for space shielding assembly.
Contacts:
Tommaso Vinci : tommaso.vinci@polytechnique.edu
Alessandra Ravasio : alessandra.ravasio@polytechnique.edu
Alessandra Benuzzi : alessandra.benuzzi-mounaix@polytechnique.edu
Some Highlights
Metallization of Shock Compressed Liquid Ammonia: At elevated pressures, ammonia is predicted to exhibit a diverse phase diagram, encompassing fluid phases with varying chemical compositions, solid phases, and superionic phases. Experimental studies on the high-pressure behaviour of ammonia have been limited due to the challenges in preparing samples for laser-driven dynamic compression experiments. Overcoming this obstacle at LULI involved the development of a specific target and allowed to measure a new equation of state for warm dense ammonia in an unexplored regime above 3 Mbar. The research also yielded the first experimental evidence of ammonia metallization and revealed its complex dissociation mechanism. The electrical conductivity of ammonia was found to be higher than water by an order of magnitude at ∼1Mbar. This discovery has implications for reevaluating dynamo models of Uranus and Neptune magnetic fields, as existing conductivity data at lower pressures suggested the opposite trend [A. Ravasio et al. Phys. Rev. Lett. (2021)] . The results have been highlighted by CNRS: https://www.inp.cnrs.fr/fr/cnrsinfo/comment-etudier-lammoniac-tres-haute-temperature-et-tres-haute-pression-pour-mieux
Melting of Superionic Ammonia at Planetary Conditions: Superionic phases, characterised by solid lattices with freely diffusing lighter ions, may exist in the interiors of ice giant planets like Uranus and Neptune, impacting their interior structure, dynamics, and magnetic fields. Accessing conditions conducive to these phases required a combination of static compression in a diamond anvil cell and dynamic shock loading. At LULI, this technique was successfully adapted to medium-size kJ laser facilities like LULI2000, enabling the exploration of relevant conditions in ammonia. The findings revealed that superionic ammonia melts at lower temperatures than water above 1Mbar, influencing the melting of stable water-ammonia mixtures. This research provides critical insights into the divergent behaviours of ammonia and water at high pressures, crucial for estimating the extent of the dynamo region in ice giants [J.-A Hernandez et al. Nat. Phys. (2023)] and highlighted by CNRS: https://www.inp.cnrs.fr/fr/cnrsinfo/de-lammoniac-superionique-cree-et-analyse-en-laboratoire