PHYHDEL (PHYsique de la matière à Haute Densité d'Energie par Laser)
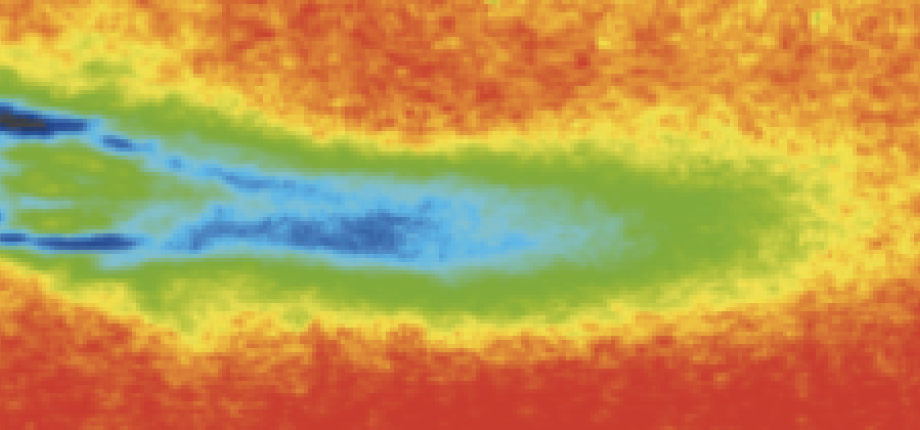
High Energy Density Physics is the scientific framework of the PHYHDEL activities. It refers to energy densities exceeding 1011 J/m3, or to pressures above 1 Mbar, and covers many topics that could be adressed with high-energy lasers and/or pulsed-power devices, thus displaying a variety of challenges for the plasma community, recently identified by the US and Japan Academies of Sciences [Frontiers in High Energy Density Physics: the X-Games of Contemporary Science & Plasma Science: Advancing Knowledge in the National Interest]. Among possible topics in this field, the PHYHDEL group research activities are centered on five themes.
Research topics
Warm Dense Matter (WDM) physics
The so-called warm dense matter (WDM) is a state of matter which lies at the frontiers between condensed matter and plasma physics. Typically, the density ranges from solid density up to 10 times solid density and temperatures vary from 0.1 to 100 eV . In this regime, matter is mostly degenerate, strongly coupled and non-ideal. The behavior and physical properties of WDM, fundamental for various branches of physics including planetology and Inertial Confinement Fusion, are consequently non trivial to simulate either theoretically, numerically or experimentally.
The Warm Dense Matter physics is a central topic of the PHYHDEL group which has been pioneer in setting a reliable method to measure equation of state (EOS) of highly compressed matter by laser-driven shock waves. These measurements were based on visible diagnostics. In the last few years, the team made an important effort to develop and couple X-ray diagnostics (X-ray Thompson scattering, XANES, X-ray diffraction) to laser-driven shocked material to obtain directly microscopic information (electronic and ionic structural changes, phase transitions, etc…).
Planetary physics
Important incertitudes in planetary models are the properties of planet constituting materials at conditions of planetary mantles and cores. For the earth, pressures reach up to 4 Mbar and temperatures up to 7000 K, giant earth-like planets can reach 15 Mbar and 15000 K. The materials of interest are iron (constituting the core), silicates and oxides (constituting the mantel) for earth like planets, hydrogen, helium, methane and water are interesting for other planets.
Having demonstrated the utility of high energy lasers to generate extremely high pressures (exceeding ten's of TPa) we opened a new path called isentropic compression to achieve the actual conditions of planetary interiors. Indeed, some fundamental questions related to the formation of planets remain open and lasers are one possible way to obtain answers. To this end, two dedicated ANR (SECHEL 2004-2007 and Planetlab 2012-2016) have been accepted. This work led to experiments on the LIL laser where a pressure up to 1 TPa has been achieved in an iron and quartz targets. The main goal of Planetlab is establish benchmarking values for the equations of states, melting curves and the transport properties in the Fe-Si-Mg-O-S complex system existing inside core of giant planets and super earths.
More recently we started another experimental program on H2O/CH4/NH3 mixtures with applications in planetology (ANR POMPEI 2016-19). Despite being a key planetary component, the states of matter, equations of state, chemical and transport properties of the H2O/CH4/NH3 system are basically unknown at planetary interiors conditions. This leaves several lacunae in our understanding of our icy giant planets, Uranus and Neptune, in which ice comprises two thirds of their mass. A triennal experimental program has been accepted at LULI 2000.
Phase transition dynamics and material science
A fundamental question for material science is the behavior of matter under high deformation rates. The rearrangement of the atoms on the crystal lattice at a phase transition and the elastic-plastic phase transition are typical examples. Many of these transitions occur on the ns time scale, which is comparable with length of the laser pulse. Laser-driven compression allows thus studying dynamic effects on phase transitions, not accessible in static compression. Using laser-driven ramp compression, we could measure the relaxation time for the crystalline restructuration of iron.
X-ray sources development & applications
Short pulse laser driven X-ray sources are an important diagnostic tool for HEDP, WDM, and pre-ignition experiments. Radiography, diffraction, scattering and absorption spectroscopy offer various possibilities to characterize basic plasma parameters like density and temperature as well as the structure of matter. All these diagnostics require powerful x-ray sources, delivering photons in the range of a few keV up to 100 keV. Additional requirements are temporal resolution (10-100 ps), spectral shape (monochromatic for diffraction or flat for absorption) and source size (down to few µm). All those diagnostics are also requiring a large number of photons, thus the x-rays must be produced with high energy laser systems. In order to prepare experiments combining a high-energy long-pulse laser to compress and heat a sample and a short-pulse laser to drive the x-ray source for diagnostics purpose, we performed a number of experimental campaigns to optimize the source characteristics and test experimental setups.
Laboratory astrophysics
We are developing for several years a growing activity in the field of laboratory astrophysics, which is booming in the world especially since the advent of a new class of lasers such as OMEGA-EP (Rochester), LIL & LMJ (Cesta), NIF (Livermore). Available energy shows that conditions close to astrophysical situations through scaling laws are accessible. Medium lasers, as LULI2000 (LULI), are very complementary to the larger facilities regarding their flexibility and accessibility.
We pursued our studies in this area on radiative shocks, plasma jets and began two new activities: cataclysmic variables & magnetic field generation. All these works on laboratory astrophysics are carried out in close collaboration with several groups worldwide. We also participate in an international consortium (ACSEL) on collisionless shocks and magnetic fields generation in the perspective of NIF shots occurring next year.
Team Composition |
Tommaso VINCI Bruno ALBERTAZZI Alessandra BENUZZI-MOUNAIX Mandy BETHKENHAGEN Michel KOENIG Victor PELLIER Alessandra RAVASIO Angelos TRIANTAFYLLIDIS Nathan VAUDRY Mathieu WEBER |